By Mimi Martinez
Polyethylene is one of the most versatile and widely used plastics globally[i], thanks to its many benefits and diverse applications. It boasts high tolerance, withstanding temperatures as high as 266°F, and excels in handling high-stress loads. Polyethylene is also highly resistant to abrasion, impact, and corrosion, making it ideal for industries such as automotive, medical, and food packaging. Its robustness and adaptability extend to various other fields, offering substantial utility in both everyday products and specialized applications[ii].
However, despite its widespread use, polyethylene recycling is challenging due to the various additives mixed with the plastic during production. Additives such as stabilizers, flame retardants, plasticizers, and pigments are incorporated to enhance specific properties like flexibility, durability, and color[iii]. These additives can interfere with the recycling process, making it difficult to separate the polyethylene from the contaminants[iv]. As a result, traditional recycling methods may not effectively recover the polymer, and the quality of the recycled material is often compromised.
Finding recyclable alternatives to polyethylene plastics that still keep their useful properties is essential in helping industries that use polyethylene make their supply chains, and product life cycles more sustainable. One solution gaining attention is using earth-abundant manganese catalysts. This process changes plant-based alcohols into polyethylene-like materials, offering similar performance while aiming for complete recyclability. Currently, recycling methods mainly use heat-based processes like pyrolysis or steam cracking, thermal processes that break down large molecules into smaller ones. These methods only recycle a small portion of the material and produce a lot of CO2 emissions in the process[v].
A significant advancement in sustainable materials has been made with polyethylene-like plastics that can fully break down into their original components under mild conditions. This was achieved through the creation of polyethylene-like materials through the chemical bonding between atoms from alcohol and carboxylic acid groups. These linkages or bonds act as designed breaking points while still preserving long methylene sequences that maintain the desirable properties of the new polymer.
The introduction of a streamlined method that utilizes the earth-abundant element manganese (feature in picture) catalysts that allows for the direct polymerization of bio-derived organic compounds without the need for a precise ratio of substance control involved in a chemical reaction or ensuring that all reactants are consumed without any leftover. The use of inexpensive manganese makes the process commercially viable[vi], and the system can produce materials with molecular weights comparable to commercial polyethylenes used in everyday applications.
The versatility of this approach comes from its ability to incorporate both linear and branched monomers. The linear components provide crystallinity and mechanical strength, while the branched segments allow for tuning properties such as elasticity and adhesion, similar to conventional polyethylene properties. Additionally, the inclusion of sulfur in the branched segments enhances the material's adhesion to metal surfaces, matching the performance of commercial adhesives. This highlights how strategic molecular design can provide additional functionalities beyond simply mimicking polyethylene’s conventional properties.
Using the same manganese catalyst, selective polymer breakdowns have a monomer recovery rate of up to 99%, even in the presence of commercial plastics and additives[vii]. This selective process targets specific polymers, allowing them to completely break down at a relatively low temperature of 110°C. Contaminants like polyethylene terephthalate (PET) remain intact, making it easier to separate them from the desired materials. The recovered monomers can then be isolated through crystallization and repolymerized, or polymer building, without significant property loss, demonstrating a closed-loop recycling process. The system has also been shown to work effectively at larger scales (10–30 g) and can handle dyed materials and various additives, showing great potential for industrial use.
As this emerging green chemistry system technology improves the possibilities for industry recycling and sustainability, there are still development opportunities that remain to be addressed for further progress. One key challenge is reducing catalyst loadings while maintaining high molecular weights, which is vital for several components of the system’s economic viability. The development of more active catalysts and simpler systems that can operate at lower temperatures and with reduced catalyst loadings could improve the process significantly as well. Additionally, the moderate thermal properties of the polymers may limit their use in some high-temperature applications. However, the exceptional recyclability of the material could make this trade-off acceptable. It has also been suggested[viii] that future research focus on optimizing the repolymerization process to ensure consistent molecular weights over multiple cycles, as well as expanding studies on moisture sensitivity and long-term performance, particularly when commercial additives are present. These steps will help enhance the economic and practical feasibility of this recycling method, pushing it closer to large-scale, industrial applications.
[ii] https://www.hpmanufacturing.com/unveiling-the-versatile-properties-of-polyethylene-thermoplastic/#:~:text=Agricultural%20applications%20for%20greenhouses%20and,to%20chemicals%2C%20corrosion%20and%20moisture
[iv] https://www.nature.com/articles/s44296-024-00024-w#:~:text=The%20complexity%20and%20diversity%20of,to%20landfills%20or%20incinerators19.
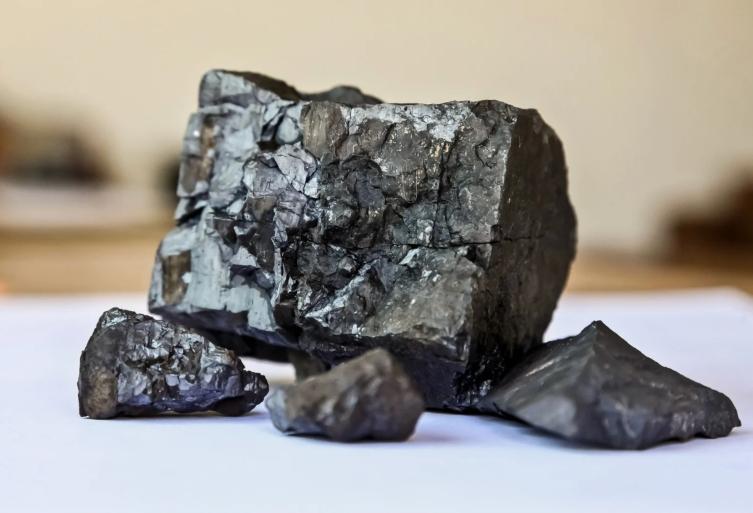