By Mimi Martinez
Recycling lithium-ion batteries nearing the end of their life cycle is crucial for both resource conservation and environmental sustainability[i]. The intensive extraction of minerals like lithium and cobalt often leads to habitat destruction, deforestation, soil erosion, and water pollution[ii]. The development of a water electrolysis-induced separation approach, which uses H2 or O2 gas bubbling to efficiently separate conductor materials from current collectors, presents a promising solution to current challenges in lithium-ion battery recycling. This process achieves 99.5% material recovery while minimizing metal impurities, helping to address existing gaps in material recycling and improving the overall sustainability of recycling methods[iii].
In order to appreciate the importance of this technology, it's helpful to first understand the structure of a lithium-ion battery. Lithium-ion batteries consist of several key components: the anode, cathode, separator, and electrolyte solution. The anode, which is the positively charged conductor, allows electrons to leave a device during use. The cathode, a negatively charged conductor, is where electrons enter the device. A separator is a thin, porous membrane that physically separates the anode and cathode, ensuring proper function while preventing direct contact. Additionally, a solution supports the movement of lithium ions between the cathode and anode during charging and discharging cycles.
The materials typically used to make the anode include metallic lithium, graphitic carbon, synthetic graphite, lithium titanate, tin-based alloys, and silicon-based compounds. For the cathode, materials such as lithium manganese oxide, lithium cobalt oxide, lithium nickel cobalt manganese oxide, and lithium iron phosphate are commonly used. Electrolytes in these batteries often include lithium slat compounds. Other minor components, such as binders, flame retardants, gel precursors, and electrolyte solvents, are also integral to the battery’s construction[iv].
The current methods for lithium-ion batteries recycling include several separation techniques, such as high-temperature treatments, chemical processes using acid or alkali solutions, and organic solvent binder dissolution. While these methods have proven effective, they come with significant challenges. High-temperature processes release harmful gases, chemical treatments require extensive post-processing to remove metal ions and manage wastewater, and organic solvents present toxicity risks and lengthy processing times. These issues, including high energy consumption and the use of hazardous chemicals, limit the ability to scale these methods for large-scale industrial use.
A recent study published in Nature introduces a new, rapid, and efficient method known as water electrolysis-induced separation (WES) for extracting cathode and anode conductors from spent batteries and manufacturing scraps [v]. This method leverages the gases produced during water splitting to enable quick separation, completing the process in just seconds to a few minutes. The oxygen evolution reaction (OER) separation allows for effective lithium extraction from lithium iron phosphate, making this approach suitable for materials with severely damaged crystal structures. The resulting iron(III) phosphate can be used as a precursor for synthesizing lithium iron phosphate batteries.
The WES method offers several advantages, including high material purity, excellent recovery rates, low energy consumption, and strong environmental benefits. The method efficiently recycles active materials and current collectors from spent lithium iron phosphate batteries and scraps. The conductor materials recovered through WES maintain their structure and can be reused in the main type of battery conductor, dry electrode manufacturing, eliminating the need for hazardous solvents like N-methyl pyrrolidone (NMP) typically used in wet electrode methods.
The efficient mixing of active materials with conductive agents in the black mass lowers the energy required for the mixing process, enhancing the method’s compatibility with the production of the dry electrodes. The remanufactured dry electrodes display electrochemical performance similar to those of new electrodes.
These advancements are significant because they address emerging market needs. Graphite recycling, once undervalued, has become increasingly important due to growing market demand and higher prices. Similarly, while lithium iron phosphate is often considered less profitable to recycle that other battry components, its growing market significance makes it increasingly important. In 2023, lithium iron phosphate production reached around 1.65 million tons, accounting for approximately 66% of the cathode materials market in China, driven by the demand for electric vehicles (EVs) and battery energy storage systems (BESS). Its safety, stability, and cost-effectiveness suggest this trend will continue. Given the environmental risks associated with lithium iron phosphate waste and the depletion of lithium resources, recycling lithium iron phosphate batteries is essential for ensuring the sustainable growth of the battery industry.
As the main anode material in almost all commercial lithium-ion batteries, graphite also makes up 10-15% of the total cost and 10-21% of the battery’s weight, contributing to substantial waste and environmental impact. Recovering graphite from spent lithium-ion batteries is essential for environmental sustainability and provides a valuable material source. This dual benefit makes graphite recycling a key part of advancing a circular economy within the battery industry.
In addition to spent batteries, large amounts of battery materials come from manufacturing waste, such as conductor scraps and rejected pieces. These materials make up about 5% of total materials in advanced production lines and more than 30% in less efficient lines. Unlike cathodes from used batteries, these discarded materials maintain their original crystal structures, making them easier to recycle[vi]. As lithium-ion batteries production increases, the importance of these materials grows. WES offers a promising solution to meet the growing demand for efficient recycling, ensuring the recovery of valuable materials while minimizing environmental impact and supporting the shift towards a more sustainable and circular economy in the battery industry.
[i] https://www.nature.com/articles/s41893-025-01539-3
[ii] https://batteriesinc.net/exploring-the-environmental-impact-and-sustainability-of-lithium-batteries/#:~:text=Battery%20recycling%20plays%20a%20vital,landfills%20or%20polluting%20our%20ecosystems.
[iii] https://www.nature.com/articles/s41893-025-01539-3
[iv]https://www.sciencedirect.com/science/article/pii/S2589299118300533#:~:text=Lithium%2Dion%20batteries%20comprise%20of,and%20electrolyte%20solvent%20%5B1%5D.
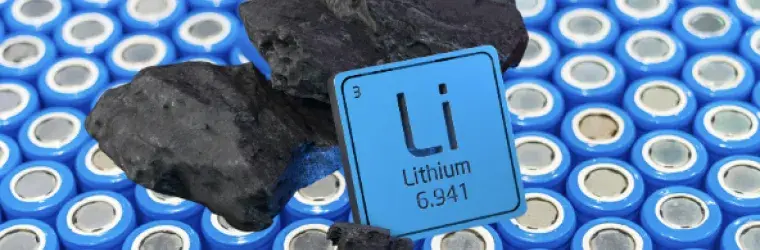